December 7, 2022
Unclassified: Labsphere White Paper
October 31, 2019
Top-of-Atmosphere Reflectance Calibration of Satellite and Airborne Sensor Systems Using FLARE Vicarious Calibration Network
…
Company Information: | Labsphere, Inc. |
---|---|
231 Shaker St. | |
North Sutton, NH 03260 USA | |
www.labsphere.com | |
Phone: (603) 927-4266 | |
Fax: (6003) 927-4694 |
Point of Contact: | Christopher Durell |
---|---|
Cell: 858-141-1885 | |
Email: cdurell@labsphere.com |
UNCLASSIFIED
INTRODUCTION
This paper introduces a new capability for performing the vicarious radiometric calibration of high, medium and low spatial resolution sensors. The SPecular Array Radiometric Calibration (SPARC) method employs convex mirrors to create two arrays of calibration targets for deriving absolute calibration coefficients of Earth remote sensing systems in the solar reflective spectrum. The first is an array of single mirrors used to oversample the sensor’s point spread function (PSF) providing necessary spatial quality information needed to perform the radiometric calibration of a sensor when viewing small targets. The second is a set of panels consisting of multiple mirrors designed to stimulate detector response with known at-sensor irradiance traceable to the exo-atmospheric solar spectral constant. The combination of these arrays with a targeting station is the basis for a new, on-demand commercial calibration network called FLARE.
SPARC METHOD – AN INNOVATION IN CALIBRATION
Current best practice for verifying absolute radiometric requirements of remote sensing imaging systems is always based on viewing a traceable extended source that uniformly illuminates many if not all detectors on the focal plane. This holds true whether the calibration images are recorded in pre-flight testing or in-flight viewing on-board or vicarious sources. Illuminating a large number of detectors establishes a mean response to the calibration radiance with high precision and averages out the blurring effects induced by the sensor’s optical system. The procedure derives radiometric gain coefficients with uncertainties that can be maintained through the image processing chain to higher level products but only for extended uniform scenes for which the response is unaffected by the sensor’s image quality performance.
Many targets of interest are small, typically subpixel up to a few pixels in diameter. As a result, the spatial performance of the sensor and any pixel resampling performed in the processing chain can become a significant error contributor to the radiometric knowledge of small targets in the scene. This leads to several factors to be accounted for in the accuracy and traceability book-keeping specific to small targets:
1.) Non-uniform energy-on-detector sensitivity: In this effect, the shape of the small target image profile projected on the focal plane detectors produces a high contrast relative to the scene background in which a single detector may ultimately respond differently because of high spatial frequency illumination gradients across the detector not present when under calibration.
2.) Sensor blurring: With sensor blurring, the energy originating geometrically within the projected ground location of a single detector, containing the small target, becomes recorded by adjacent detectors outside the target area because of the system point-spread function (PSF). This induces a probabilistic uncertainty in the location of the signal’s origin and, thus, also the target radiance without some a priori knowledge of the targets shape and size.
3.) Effects of post processing on image quality: In post processing, band-to-band registration and geometric calibration apply resampling methods that ultimately introduce increased radiometric uncertainty. The result is additional spatial smearing of information between the small target and its background specific to the resampling kernel and routine used to redefine individual pixel digital values, size and orientation.
Due to these factors, there is a need to develop a comprehensive radiometric error propagation analysis process for small targets that can benefit by introducing vicarious ground references that reveal and validate these artifacts within image data collected under operational conditions.
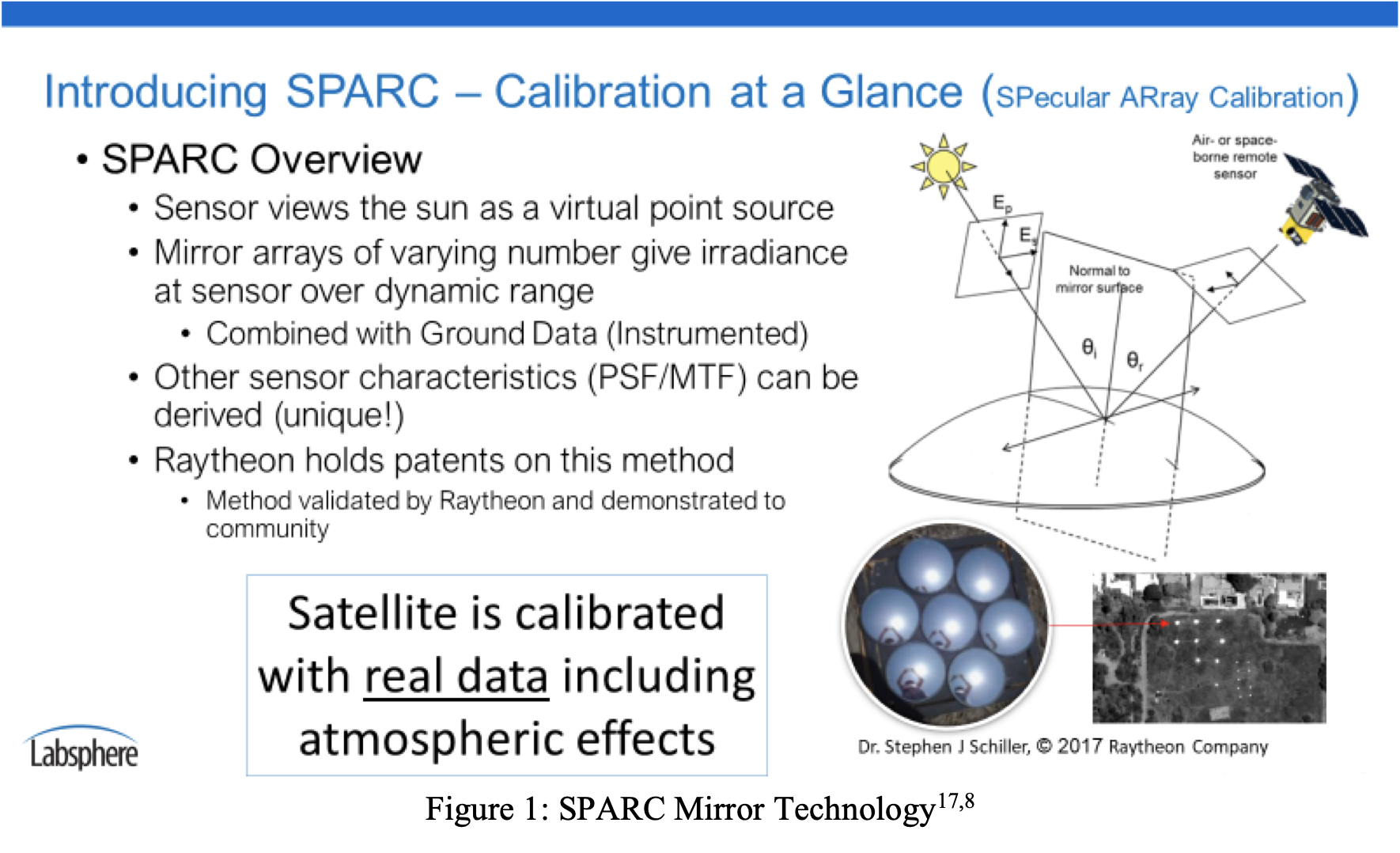
The vicarious calibration technique known as the Specular Array Radiometric Calibration (SPARC) method is demonstrated to provide a highly flexible and robust approach for characterizing the performance of a sensor imaging small targets. Its general use is shown in Figure 1. This technology is the invention of Dr. Stephen Schiller of Raytheon and has a well-documented record of published performance (see Appendix 1). SPARC delivers small intensity reference targets, made from a collection of facets consisting of convex mirrors, which can be built to any size, shape, brightness or spectral composition with accurate radiometric traceability to the solar radiometric scale. These types of synthetic targets may be a dynamic replacement for naturally observed phenomena (PICS sites, deserts, etc).
THE FLARE NETWORK – ON DEMAND LOW COST CALIBRATION
Labsphere has created a new automated calibration network called FLARE based on the SPARC mirrors and their established radiometric technique. FLARE is an acronym that stands for Field, Line- of-sight Automated Radiance Exposure. The benefit of FLARE is a point-able system with improved radiometric performance knowledge compared to other in-flight vicarious techniques through reduced uncertainties in target reflectance, atmospheric effects, and temporal variability. The only ground truth needed is the measurement of atmospheric transmittance (although upwelling and downwelling radiance, surround reflectance, and mirror characteristics are also collected by FLARE stations). These mirror arrays are sub-pixel size. The simplification of calibration targets and ground truth collection in the FLARE Network makes the deployment more cost effective and/or portable. FLARE creates the opportunity to strategically locate and imbed spectral, spatial, and radiometric targets at study sites providing references that improve a sensor’s interactivity as a phenomenological tool. This technology is
patented by Raytheon Inc. and licensed to Labsphere, Inc. in order to create a commercially available network for calibration.
The FLARE Network concept is an innovative, automated, on-demand, ground-based radiometric calibration station for in-flight sensors. These stations will track and point to sensor (satellite/airborne) locations and provide absolute ground-truth calibrations and direct evaluation of image quality. They hold the promise to provide standardization among imaging satellites and Analysis Ready Data (ARD) at a first level of image capture (L0 or L1). An extended network of these systems will be the basis for fractional-cost calibrations, digital subscription services and worldwide common calibration operation for all airborne and satellite imagers as shown in Figures 2 and 3.
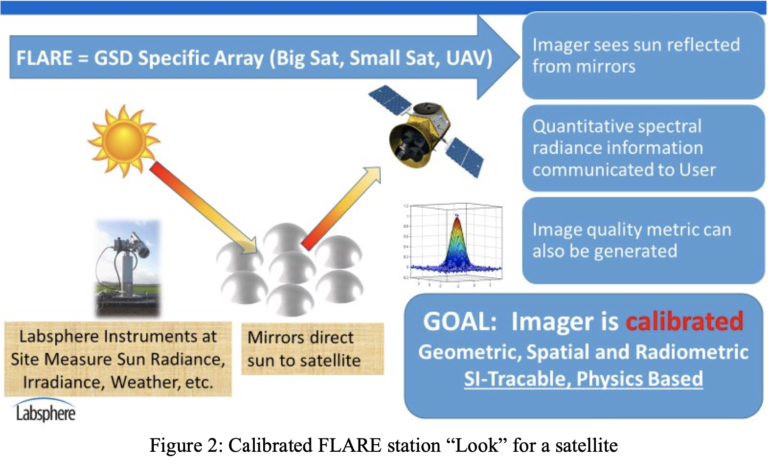
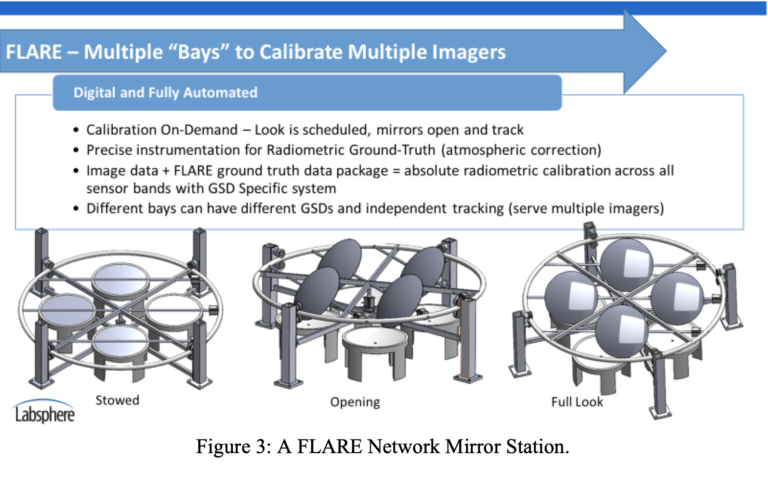
Initial development of the commercial FLARE network is targeting an Alpha system for evaluation by university and commercial partners in early CY2020, which will be followed by a more feature-rich Beta system by Summer of 2020 that includes the complete subscription-based revenue platform. The network will seek commercial imaging and Government customers who are looking for a cost-controlled, on-demand, traceable calibration system for their satellite and airborne instruments. The network will expand as quickly as the market uptake of the concept allows. A model of this expansion is shown in Figure 4. The intent of this information is to seek customers as a faster means for expanding this capable network to speed the possibility of Analysis Ready Data from all sensor platforms.
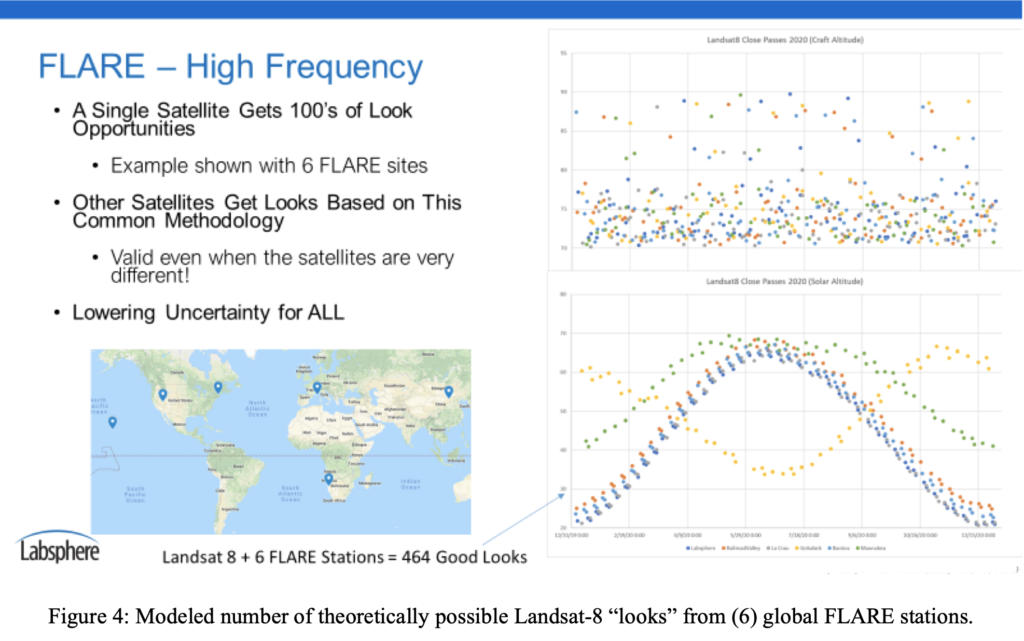
ENHANCING CURRENT VICARIOUS CALIBRATION METHODS WITH FLARE
The FLARE network will offer a breakthrough technology to the satellite and airborne imagery community in many ways:
• Tailored to imagers, FOVs & instruments (GSD, angle independent, & bands)
• Agile system configuration, innovative uses and universal placement capability
• Tied to “Big Satellite” methods: Same or better traceable uncertainty & quality metrics
• Traceably link satellite (lower resolution) to higher resolution airborne, UAV high resolution “under-fly” data.
• Provide on-demand calibration services: when and where needed…and as often as needed
• Subscription and per-look business model: Fractional & controlled cost versus conventional calibration methods.
• Enables Analysis Ready Data as close as possible to the initial image processing levels.
Additionally, FLARE can offer some revolutionary capability to solve problems that are not currently addressable by common techniques. A discussion of specific problems and solutions is embedded in Table 1 through Table 5 below.
PROBLEM AREA | CURRENT VICARIOUS FLARE SOLUTION | CITATION OR STATUS |
---|---|---|
Limited by GSD, & Bands | FLARE stations can have multiple mirror sets so that looks are GSD Specific. The mirrors are broadband, characterized and have a spectrally neutral coating (e.g. aluminum) so that they are only relaying the measured solar spectrum. | 17, 14, 6 |
One-site, one type of calibration | FLARE sites can have multiple mirror bays with independent controls. Different mirrors in different bays can allow different calibrations for GSDs or polarization or other factors. Or, different mirrors could simultaneously or sequentially track different targets imagers providing a common calibration. | 15, 12, 6 Pending – rapid sequential testing with different platforms. |
Mis-matched Radiometry and FOV | FLARE is tailored data to each GSD and is a subpixel point source – this removes the dependence of each satellites’ GSD and FOV (and target BRDF). Any mismatching of bands due to angular and spatial registration is readily apparent in FLARE images and can be quantified and corrected. | 17, 14, 7 |
Follows the Big Satellites for SNO | Since the FLARE systems can point and can be placed at any advantageous location, and utilize TOA sunlight, a Simultaneous Nadir Overpass (SNO) is not necessarily needed with Big Satellites to establish traceable measurements for small satellites. Small satellites could choose orbits and calibration scenarios to achieve objectives without being tied to big satellite orbits. | 17, 14, 13, 8, 7, 6 Pending – test with campaigns with small satellites. |
Limited & Large Sites | Current calibration sites are large deserts and natural areas to accommodate the large pixel sizes of the big satellites. FLARE synthetic targets can be placed in any location fields, parking lots, tops of buildings, mountains. Any measured semi-uniform surface has the potential to be a calibration site with a static or mobile FLARE station. | 17, 14, 8, 7, 6 Pending – test with Alpha and Beta units & campaigns w/small satellites. |
Government Program Dependent | FLARE is a commercially available system. It is a going-concern based on its own utility and economic merits as a paid service to its customers. It is not inherently reliant on government funding sources. | FLARE commercialized services to start in CY2020. |
Table 1: Inherent problems for current calibration methods and how FLARE can help along with citations of reference work.
PROBLEM AREA | ATMOSPHERIC AND SITUATIONAL FLARE SOLUTION | CITATION OR STATUS |
---|---|---|
Low uncertainty calibration | The atmospheric variance is usually one of the largest uncertainties in any image capture. High Altitude placement of FLARE sites to reduce atmospheric transmittance and uncertainty to fractional levels. FLARE mirrors could also be placed in space (at GEO or on other LEO satellites). | 17, 9 Pending – Planned for site placement in 2020/2021. |
Atmospheric extinction | Reducing atmospheric effects to only transmittance allows accurate atmospheric characterization using Aeronet-style instruments alone. Atmospheric values are measured not modelled. FLARE could be an expansion or possible improvement to the Aeronet readings because the full atmospheric path length is measured. | 16 Pending – FLARE can be tied to Aeronet for comparison. |
Partially cloudy days | The at-sensor signal contains only the direct solar contribution and, thus, does not require a completely clear sky, only a clear line-of- sight as defined by the mirror pointing. | 17, 14 Pending – Planned campaigns with Universities and commercial. |
Variable angle atmospheric passes | The FLARE system continuously tracks the satellite through its orbital path so that the target can be imaged anywhere along the path across the sky. Atmospheric transmittance is measured. Knowledge of the exact position in the sky at which the ground target is imaged is not necessary to obtain a calibration collect | 16, 8 Pending – Possible using tracking capability of FLARE and pointing satellites. |
Mobility | Current calibration sites are large and immobile. FLARE mirror sets and pointing stations are generally small and easy to move (size is GSD specific). They can be placed on mobile vehicles or tripods to move them where the measurements need to be made. Sites can be man-made (buildings, parking lots) or natural, as long as the background is characterized and/or low reflectance. | 8, 1 |
Table 2: Atmospheric and Situational Problems for current calibration methods and how FLARE can help along with citations of reference work.
PROBLEM AREA | SPECTRAL & SPATIAL FLARE SOLUTION | CITATION OR STATUS |
---|---|---|
Unconventional orbits | Currently small satellites generally have no on-board calibration capability and must rely on vicarious calibration methods and orbit paths of big satellites to verify image uncertainty. FLARE sites could be placed in convenient locations (permanently or temporarily) to allow low or high inclination orbits for frequent revisit or task- specific purposes. | 8, 5 Pending – Possible due to the mobility and small footprint of systems. |
BRDF of targets | If FLARE continuously tracks the satellite through its orbital path, atmospheric measurement can be extracted. The satellite would need to point back at the same point of the earth in a repeated fashion and then back at FLARE. This successive series of looks and atmospheric measurement may allow BRDF of a target to be extracted from this data set. | 17, 14, 8 Pending – Possible using tracking capability of FLARE and satellite pointing. |
Large footprint targets | FLARE targets, being intensity sources rather than radiance sources, can be kept small and do not require multiple pixels for calibration | 17, 14, 13, 8, 7, 5, 4 |
Polarization | Mirrors with known polarization states (S, P, both or other) can be introduced into the FLARE stations by the mirror surfaces. Look angle orientation and angle of incidence of the sun on the mirror can be controlled independently to provide different degrees of linear polarization in different states. | 15 Pending – test with ocean clients and other sites. |
Natural site spectral variation | FLARE sites are synthetic targets that provide engineered spectral responses. Synthetic sites will reduce variation in imagers response and band-to-band uncertainty. | 17, 14, 13, 8, 7, 6 |
Spectral signatures | Multiple mirrors are usually populated in a bay with one coating. It each mirror had a bandpass (coating) then the summation radiance signature of the mirrors would be a convolution of the independent mirror bands. This capability creates tunable mirror spectral signatures that can be designed to emulated reflected signatures or unique task identification signatures. | 4, 3 |
Table 3: Spatial and Spectral Problems for current calibration methods and how FLARE can help along with citations of reference work:
PROBLEM AREA | IMAGING & INTER-CALIBRATION FLARE SOLUTION | CITATION OR STATUS |
---|---|---|
Ocean leaving radiance | These mirror sites could be placed onto structures (like oil platforms) that provide a bright target with dynamic range control on top of a large dark background (ocean). The line-of-sight path provides extremely well understood atmospheric information. Additionally, these mirrors have shown to be able to be used on water and still provide point sources – even with wave action – due to spherical shape. Polarization capability with different mirrors can be installed. Additionally, mirrors even the ability to submerge for bathymetry radiance and you have a complete tool set that may be able to finally solve the Ocean Leaving Radiance problem – or at least provide a well understood uncertainty that is not possible with today’s methods. | 15, 4, 2 Pending – Creation of large-scale sites for ocean programs. like PACE or GLIMR. |
PSF, MTF and Empirical Line Method | FLARE targets provide a true point source with calibrated radiance. Arrays or patterns of these targets can provide PSF, MTF or other image related quality information. Using different numbers of mirrors in different clusters, captured in a single image, provides different brightness points to create an accurate linear calibration over the full dynamic range of the sensor. | 17, 8, 1 |
Under-Fly and Over- Fly with different instruments. | FLARE stations can have multiple mirror bays with independent controls. Different mirrors in different bays allow different calibrations for GSDs or polarization or other factors. Or, different mirrors could simultaneously track different targets imagers at the same time providing a common calibration. Response of the sensors is independent of altitude as long as the GSDs are similar. | 15, 12, 6 Pending – Tests with small sat and UAV providers. |
GEO & LEO intercalibration | To a GEO imager, a FLARE system would be like a constant solar reference, which means the GEO imager constantly monitors the solar transmittance providing real-time atmospheric and radiance data. As a result, GEO sites observing these FLARE sites could then be linked or correlated to simultaneous LEO overpasses and the GEO data could calibrate the LEO satellites. Conversely, telescopes on earth could use a SPARC at near-GEO to cross calibrate with each other radiometrically. Or, LEO satellites with FLARE mirrors might be able to cross-calibrate each other. | 14, 9 Pending – Testing and research needs to be done but theoretically possible. |
AM/PM & Night Calibration | The FLARE systems are only reliant on having a constant point source of radiance. Solar looks at the beginning and end of the day could be possible to provide atmospheric information or dawn/dust spectral calibrations. Additionally at night, the moon can be used to calibrate night vision or day/night band type systems. | Pending – Radiometrically possible, has not be tested. |
Table 4: Imaging and Inter-Calibration problems for current calibration methods and how FLARE can help along with citations of reference work.
PROBLEM AREA | COMMERCIAL AND ECONOMIC FLARE SOLUTION | CITATION OR STATUS |
---|---|---|
Maintain Calibration Team or calibration campaigns | Traceable calibration is available on an as-needed basis and costs can be controlled to suit constellation or individual satellite needs. High precision and repeatability allow for rapid and frequent on-orbit validation. | Pending – Commercialized subscription service and on-demand “looks” pricing. |
Maintain Image Processing Team (IPT) | Frequent calibrated images with instrument specific GSD band independence and FOV information will lead to analysis ready data on each overpass and less processing time and resources. | Pending – studies with current providers to show cost reduction and efficient ARD products. |
There is no ARD “Standard” | Highly defined and agile FLARE methodology provides a basis to establish an ARD standard or multiple ARD definitions. Availability and agile configuration of FLARE network and systems will enable affordable and easy ARD standard compliance. | Pending – Work with CEOS and other Government organizations to help determine a feasible ARD standard. |
Low frequency of valid opportunities | Frequency of FLARE looks could drive down individual systemic uncertainty just through gross statistics. Frequency and trending will enable innovation and new capabilities. | Pending – FLARE commercialization and utilization studies. |
Human intervention | The amount of data today requires full automation (AI and ML) to achieve the data’s value potential. Even with free Big Satellite calibrated data, today, significant human intervention on the imagery and calibration quality is required. FLARE will enable fully automated and cloud-based services with verified performance with minimal human resource intervention. | Pending – FLARE use study on reduction of human intervention and data quality. |
Table 5: Commercial and Economic Problems for current calibration methods and how FLARE can help.
CONCLUSION
The amount of airborne and satellite data available today is expanding exponentially, but its inherent quality and radiometric uncertainty is not matching pace. Better data is needed, not just more data. Accelerating the quality of the data demands a new breakthrough system of a high-frequency, reliable, and practical system calibration. SPARC is a proven technical solution for calibration. FLARE systems are synthetic targets that offer a huge range of engineered, stable and calibratable solutions that current natural sites methods do not. Rapid expansion of the FLARE network will result in automation of calibration and inherently better image quality and data. As the world grapples with the “big data” era, fundamentally improving the ARD baseline of that data will enhance the inherent value to customers. Better data will save valuable man hours, enhance data extraction techniques (AI and ML) and allow data-driven systems to make better decisions and develop new capabilities. The FLARE network is the revolutionary tool needed to advance the quality of the world’s aerial and satellite image products.
APPENDIX 1: LIST OF TECHNICAL CITATIONS
1 Schiller, S.J., “Demonstration of the Mirror-based Empirical Line Method (MELM)” 18th Annual Joint Agency Commercial Imagery Evaluation (JACIE) Workshop, Reston, Virginia, Sept. 24-26, 2019Schiller, S.J., “Demonstration of the Mirror-based Empirical Line Method (MELM)” 18th Annual Joint Agency Commercial Imagery Evaluation (JACIE) Workshop, Reston, Virginia, Sept. 24-26, 2019.
2 Ortiz, Joseph, et. al., Evaluating visible derivative spectroscopy by varimax-rotated, principal component analysis of aerial hyperspectral images from the western basin of Lake Erie, Journal of Great Lakes Research, Volume 45, Issue 3, Pages 522- 535, February 2019.
3 Silny J., and Schiller, S., Method and system for spectral calibration of a remote sensing sensor and a synthetic target having a tunable spectral composition, US8507843, United States Patent and Trademark Office, 13 Aug. 2013.
4 Ortiz, Joseph, et. al., Intercomparison of Approaches to the Empirical Line Method for Vicarious Hyperspectral Reflectance Calibration, Frontiers in Marine Science 4:296, August 2017.
5 Stephen Schiller, John Silny and Marcus Teter “Comprehensive Vicarious Calibration and Characterization of a Small Satellite Constellation Using the Specular Array Radiometric Calibration (SPARC) Method”, Proceedings of the 31st Annual AIAA/USU Conference on Small Satellites, 2017, Logan Utah.
6 Stephen J. Schiller and John Silny, 2016 GOINT Electro-Optical Technical Symposium (GEOTS), National Geospatial- Intelligence Agency, Springfield, VA. “Characterizing The Small Target Radiometric Performance of Remote Sensing Imaging Systems”, Dec 12, 2016.
7 Schiller, S., and Silny J., “Using Vicarious Calibration to Evaluate Small Target Radiometry” 25th Annual Meeting on Characterization and Radiometric Calibration for Remote Sensing (CALCON), Logan, Utah, 2016.
8 Schiller, S. and Silny, J. “Application Of The Specular Array Radiometric Calibration (SPARC) Method For The Vicarious Calibration Of Landsat Sensors”, 15th Annual Joint Agency Commercial Imagery Evaluation (JACIE) Workshop, Fort Worth, Texas, April 12-14, 2016.
9 Rudy, R.J., et. al., “Absolute Radiometric Calibration Using a Solar Reflector in Near-Geosynchronous Orbit” 24th Annual Meeting on Characterization and Radiometric Calibration for Remote Sensing (CALCON), Logan, Utah, 2015.
10 Stephen J. Schiller, John Coogan, David McCoy and Robert Opalecky, 2013 GOINT Electro-Optical Technical Symposium (GEOTS), National Geospatial-Intelligence Agency, Springfield, VA. “A Fresh Look At Small Target Radiometry: Measurement of Net Radiated Power”.
11 Schiller, S. and Silny, J. “In-Flight Performance Assessment Of Imaging Systems Using The Specular Array Radiometric Calibration (SPARC) Method”, 11th Annual Joint Agency Commercial Imagery Evaluation (JACIE) Workshop, Fairfax, Virginia, April 18, 2012.
12 Schiller, S., Silny, J. and M. Taylor, “The Specular Array Radiometric Calibration (SPARC) Technique As A Vicarious Methodology For Accurate Intersensor Calibration” 21st Annual Meeting on Characterization and Radiometric Calibration for Remote Sensing (CALCON), Logan, Utah, 2012.
13 Specular Array Radiometric Calibration (SPARC) In-Flight Sensor Performance Assessment SAEF/SMUG Conference (Classified conference), SI Stonegate Facility, Chantilly, Virginia, June 26, 2012
14 Schiller, S.J., Specular array for radiometric calibration and method, US8158929, United States Patent and Trademark Office, 17 April 2012.
15 Silny J., and Schiller, S., Polarimetric calibration of a remote sensor, US9372119, United States Patent and Trademark Office, 21 June 2016.
16 Stephen J. Schiller, John Silny, “Measuring Atmospheric Optical Depth Directly from Satellite Imagery”, JACIE 2011 Civil Commercial Imagery Evaluation Workshop, Boulder, CO, March 29-31, 2011.
17 The Specular Array Radiometric Calibration (SPARC) method: a new approach for absolute vicarious calibration in the solar reflective spectrum, Schiller, Stephen J.; Silny, John; Remote Sensing System Engineering III. Edited by Ardanuy, Philip E.; Puschell, Jeffery J. Proceedings of the SPIE, Volume 7813, pp. 78130E-78130E-19 (2010).
18 Helder, D., Choi, T, and M. Rangaswamy, “In-flight characterization of spatial quality using point spread functions”, in Post-Launch Calibration of Satellite Sensors, Proceedings of the International Workshop on Radiometric and Geometric Calibration, December 2003, Mississippi, USA., Eds., Stanley A. Morain, Amelia M. Budge, CRC Press, 2003.